1.IntroductionThe principle of selective photothermolysis involves absorption of a spectral dose of intense laser energy at specific sites. It induces localized heating without affecting the surrounding tissues. The exposure period should be longer (or equal) to the target thermal relaxation time to obtain sufficient heating affect. The success of selective photothermolysis 1 is based on the specific wavelength, pulse length, energy fluency and spot size depending upon the optical properties of coetaneous condition. The treatment is simple when the target and tissues around it have different absorption coefficients. However, when the target and overlying area consist of the same tissues, then thermokinetic selectivity can play a vital role. It is obvious that the absorbed energy diffuses slower from larger targets than smaller targets of the same size chromophore. It is said that the pulse duration must be equal to the target cooling time but shorter than the epidermis relaxation time. This helps the epidermis to remain cool while larger targets such as leg vein or hair follicle internally heat up. However, the laser thrombolysis becomes complicated if arterial occlusions are a combination of plaque and thrombus. The initial use of continuous wave (cw) lasers resulted in thermal necrosis and even charring that further led to intense vapospasm, thrombosis and intimal hyperplasia. The cw laser was eventually replaced by pulsed excimer and Ho:yttrium–aluminum–garnet (YAG) lasers, but that caused an unwanted photochemical reaction due to breakage of carbon bonds in tissues, microcavitation, uncontrolled damage to adjacent tissues and indiscrimination between target and vessel walls in perforations. The relaxation time of thinner tissues (for example, in lungs) may be very small. The damage threshold for acute arterial thrombus and normal arterial tissue, at 480 nm, are 15 and 1500 mJ/mm 2, respectively. In view of above the pulsed dye laser, at 480 nm with 1–2 μ s duration, it is considered appropriate but even shorter durations may prove more successful. This area requires more research. 2.Choice of Pulsed LasersChoice of flash-lamp pumped dye laser (FPDL) in treatment of coetaneous port-wine stains (PWS), hypertrophic scars, vascular lesions, condyluma, rosacea, cherry birthmarks, spider leg veins, keloids, blemishes, childhood-haemangiomata, stretch marks, lip creases, angiomas, facial telangiectasia and hemangiomas indicates bright signs of progress in the field of biomedical optronics. Earlier lasers replaced by FPDL 2 3 4 include those which operate on 480 and 514 nm wavelengths. There is a general consensus on the option of flash lamp pumped dye lasers for treatment of port-wine stain birthmarks and little diversity exists in observation and opinion on postoperative results of treatment using dye lasers. For example, Alster et al.; 5 (Washington Institute of Dermatology) treated the CO 2 and Nd:YAG induced scars and cafe´-au-lait birthmarks with FPDL at 510 and 585 nm with energy fluency of 2–4 J/cm 2 without side effects such as lesional recurrence and skin textural change, etc. Rather, they report return of normal skin marking after treatment with dye lasers. However, Tan and Vinciullo 6 (Royal Perth Hospital, Australia) in general agree to the findings of Ulster et al.; but they have recorded some minor adverse affects, too. These adverse affects include scars and textural or pigmentary changes in 11 of patients that is nearly 6 more than others. Bio effects are caused by photochemical reaction or thermal processes that depend upon excessive energy fluency. They can be related to gradual gain accumulation in repetitively pulsed lasers that constitutes the core idea to be discussed in this paper. The U.S. Occupational Safety and Health Administration (OSHA) instruction publication 7 also invites further study on a curious reduction of damage threshold using repetitive short exposures. This document further comments that short pulse exposures with longer inter-pulse periods (ms) are linearly additive but their origin cannot be predicted on the basis of heat flow. Until recently long pulse low peak power lasers such as CO 2 (250 μ s), Nd:YAG (quasi-cw), Er:YAG (300 μ s) and FPDL (200–1500 μ s) were used for gynecological surgery, resurfacing, PWS, pigmented lesions, tattoos, acne and laser treated scarring. The long pulse (ms μ s) and moderately short pulse (μ s ns) laser treatments in a few cases 7 8 9 10 led to postoperative bio effects such as pigmentary disturbances, persisting erythema, edema, surface redness, bacterial and viral infections resulting in irritation or psychological harassment in treated patients and others waiting for treatment. The theory of selective photothermolysis stimulated 10 11 12 researchers to go for even shorter pulse (ns ps) lasers to avoid problems associated with long pulse or cw lasers. Fortunately, dye lasers can meet all the above requirements in a most economic manner. Dye lasers have the potential capability of producing ultrashort (a few fs), tunable pulses that make them so distinguished from other lasers. Dye lasers come in different versions and designs such as FPDL, diode pumped dye laser and distributed feedback dye laser (DFDL). As the DFDL is the cheapest source of ultrashort laser pulses, this study, to be described in this paper, has been focused on experimental investigation of DFDL output pulse characteristics regarding unintended overexposure. The objective of this paper is to show that DFDL output pulses may differ from specified pulse shape and power, therefore causing unintended biological effects. 3.Gain Accrual Caused OverexposureWhen a dye cell is pumped by the interference pattern of two beams, obtained from the same source, a temperature grating is induced on the surface of dye solution. It pumps the dye medium to provide gain and a refractive index modulated grating to supply positive feedback to cause laser action to occur. Such temperature gratings (also called gain gratings) take some time 13 to relax to the initial equilibrium temperature. If the pump pulse (Q switched laser) is replaced by a train of pulses (mode-locked laser) then the overall effective temperature depends upon the inter-pulse time of the exciting laser. It was found in the present study that succeeding pulses do contribute to the receding pulses. It will be shown that the pulse powers, shapes and energies of excitation laser (Nd:YAG) and the DFDL do not correspond to each other. The occurrence corresponds to each other but their temporal and spectral characteristics along with over pulses envelope profiles are different. A computer program was written to calculate the percentage energy retained by medium from each succeeding pulse by simulating the experimentally measured results. Simulation results showed that the gain accumulation, responsible for pulse broadening and overall power instability, becomes too sensitive for shorter cavity lengths (inter-pulse periods). However, the effect is not notable for longer inter-pulse periods. The author, in the light of Ref. 7, believes that above temperature accumulation can cause lasing of some unexpected pulses that otherwise for longer inter-pulse periods cannot lase. This is likely to cause overexposure or power instability. 4.Experimental ArrangementsTo demonstrate unintended pulse size, shape and energy deviation a frequency doubled, laboratory built passively Q switched and mode-locked, Nd:YAG laser was used to excite the solution of Rh6G in ethanol. The dye laser solution was prepared at a concentration of 10−3 M. The concentration of modulating dye (Eastman Kodak 9740) in solvent (chlorobenzene) was adjusted at a constant pump power so as to produce 20 pulses such that intensities of half of them were above half maximum. The second harmonic generation was conducted by beta barium borate crystal. The unconverted infrared (IR) was reflected out of green beam by a dielectric mirror, which had high reflectivity for 1.06 μ m at 45°. This unconverted IR pulse was used to trigger the streak camera for performing measurements. The 5 green energy reflected along with IR was used as reference signal. Complete optical circuit for this experimental setup is shown in Figure 1. The green output was reflected by a mirror and focused by a cylindrical telescope. The main green beam (0.532 μ m) was split up by a 25 mm radius 10-mm-thick dielectric beam splitter. Two mirrors FM1 and FM2 were used to finally overlap the 2-mm-long line focus of the split pump beams at dye cell. Both beams were superimposed on each other with optimum optical path difference to cause normal operation of the DFDL in Shank 14 -type configuration. The DFDL (signal) and Nd:YAG (reference) output were made to travel a distance of 8 m in air to reach the entrance slit of the streak camera. The reference and signal pulses were adjusted to appear on each using a two-channel oscilloscope and the measurements were taken by streak camera for three different adjustments of cavity length to vary the inter-pulse periods of the pumping pulses. 5.Results and DiscussionsThe streak records were taken on HP-5 films. These films were stamped with step filter array before processing. After development the negatives were scanned with a computer controlled microdensitometer. The streak records were scanned at a step of 0.05 mm and step filter arrays at a step of 2 mm. The density data were plotted as a function of Log I was plotted using a curve-fitting program. The polynomial function for intensity (I ) in terms of optical density (ρ) is shown below The intensity plot of Nd:YAG (solid) and DFDL (dotted), as shown in Figure 2, indicates that there is a visible trend of shifting of the peak pulse toward the trailing edge in DFDL profiles. To clearly inspect the data profiles a 4 ns delay was introduced in DFDL pulses. This is just to identify the overall profiles of both lasers that otherwise overlap. This delay was subsequently subtracted from the measured effective delays between both laser pulses. Several measurements were taken initially on Polaroid-47 films and later on HP-5 films for final observation. Streak records were taken at 1 or 2 ns/mm sweep speed to capture the maximum number of pulses. After calibration the nominal streak speeds were found to be 2.87±0.15 and 5.67±0.1 ns, respectively.The overall effect was the peak pulse shifting by more than an inter-modal period as shown in Figure 2. This happens due to an increase in overall accumulated temperature (hence the gain, too) in the dye medium. Variation of peak pulse shifting as function of inter-pulse period (2L/C) is shown in Figure 3. Similarly a variation of the percentage amplitude (r) retained by the gain medium (obtained through simulation) as a function of the inter-pulse period is shown in Figure 4. Figure 3Variation of delay between Nd:YAG and DFDL peaks as a function of time between two pump pulses. ![]() Figure 4Variation of percentage amplitude retained by the medium as a function of the time between the two pump pulses. ![]() It is concluded that the gain accumulation in the case of smaller 2L/C is higher than longer 2L/C. 15 The experimentally measured delays of 14.4±0.5 ns for 2 L/C =4.43 ns, 12.8±0.5 ns for 2L/C=5.93 ns and 10.9±0.5 ns for 2 L/C =8.31 ns correspond to computer calculated (assuming Gaussian profiles) r (part of the previous pulse retained by medium while the next pulse comes) of 74.2, 67.5 and 57.6, respectively. Variation of r and maximum delays between Nd:YAG and DFDL, assuming Sec 2 -type profiles, were computed to be equal to 3.89, 1.725 and 1.23, respectively, for the above mentioned inter-pulse periods. Further, it was interesting to observe that Nd:YAG pulses remain stable in length but the DFDL pulses broaden gradually. The last pulse of the total ten pulses was measured to be double the length of the first one. It cannot be a pin cushion effect as a similar effect was also observed in oscilloscopic records. It was therefore concluded that DFDL output pulses are likely to differ from the expected number and vary in size, too. This means the energy content is likely to deviate toward overexposure. This important aspect needs to be considered for precision engineering and medical applications. 6.Modeling of Temperature Phase GratingThe temperature gratings responsible for laser action in dye medium decay very slowly. If the relaxation time constant is τR then the general equation of thermal decay in dye solution as function of time t may be given by where Ao is the maximum amplitude of the temperature grating caused by any single pump pulse.At t=2L/C the above equation becomes The previous temperature grating is still diffusing and a new grating is created by the upcoming pulse. The effects (amplitude) added from the previous pulse may increase the overall temperature.Let the temperature retained be r=Ao/A at time t+2L/C. It means Eq. (3) can be rewritten as The plot of 2L/C vs r from simulation and experimental data gives the following empirical relation: After some simplification Eq. (5) may be rewritten as Theoretically, the thermal relaxation time constant of temperature grating can be calculated by where Λ is the period of grating, cP is specific heat and K is the thermal conductivity. Comparison of Eqs. (4) and (6) indicates that thermal relaxation time constant of Rh6G in ethanol at the dye concentration of 10−3 M is τR=15.8 ns, whereas calculated value using different concentration is reported 16 17 18 to be 16.5 ns for methanol and 12 ns for water.7.ConclusionsIt was shown that power, shape and energy of excitation laser (Nd:YAG) and the DFDL do not correspond to one another due to gain accumulation and pulse broadening. The occurrence corresponds to each other but their temporal and spectral characteristics are different. Simulation results showed that the gain accumulation, responsible for pulse broadening and overall power instability, becomes too sensitive for shorter cavity lengths (inter-pulse periods). However, this effect is not notable for longer inter-pulse periods. The temperature accumulation can cause lasing of some unexpected pulses that otherwise for longer inter-pulse periods cannot lase. It was therefore concluded that DFDL output pulses are likely to differ from the expected number and profile. This means the energy content is likely to deviate toward overexposure. This important aspect needs to be considered for precision engineering and medical applications. These results describe the fundamental laser and thermodynamic physics toward better understanding of excessive heat accumulation while using repetitively pulsed lasers. REFERENCES
R. R. Anderson
and
J. A. Parrish
,
“Selective photothermolysis: Precise microsurgery by selective absorption of pulsed radiation,”
Science , 220 524
–527
(1983). Google Scholar
H. J. Lowery
and
J. Zelickson
et al.;,
“Treatment of leg telangiectasia using long pulse dye lasers at 595 nm,”
Lasers Surg. Med. , 20 1
–5
(1997). Google Scholar
J. M. Garden
, and
A. D. Bakus
,
“Clinical efficacy of pulsed dye laser in the treatment of the vascular lesions,”
J. Dermatol. Surg. Oncol. , 19 321
–326
(1993). Google Scholar
S. A. Tina
and
A. K. Kurban
et al.;,
“Alteration of argon induced scars by the pulsed dye laser,”
Lasers Surg. Med. , 2 369
–373
(1993). Google Scholar
R. Ashinoff
and
R. G. Geronemus
,
“Flash-lamp pumped pulsed dye laser for port-wine stains: Earlier vs later treatments,”
J. Am. Acad. Dermatol. , 24 467
–472
(1991). Google Scholar
U. Hohenleutner
,
W. Baumler
,
S. Karper
,
S. Michel
, and
M. Landthaler
,
“Experience with the early treatment of childhood haemangiomata using the flash-lamp pumped dye laser,”
Lasers. Med. Sci. , 4 181
–183
(1996). Google Scholar
J. M. Garden
,
L. L. Pola
, and
O. T. Tan
,
“The treatment of PWS by pulsed dye laser: Analysis of pulse duration and long term therapy,”
Arch. Dermatol. , 124 889
–898
(1988). Google Scholar
H. Kogelnik
, and
C. V. Shank
,
“Simultaneous emission in periodic structures,”
Appl. Phys. Lett. , 18 153
–154
(1971). Google Scholar
V. I. Vashchuk
and
E. I. Zabello
et al.;,
“New dye laser systems with dynamic distributed feedback,”
Sov. J. Quantum Electron. , 8 859
(1978). Google Scholar
P. Y. Key
and
R. G. Harrison
,
“Bragg reflection from a phase grating induced by nonlinear optical effects in liquids,”
Sov. J. Quantum Electron. , QE-6 645
–647
(1970). Google Scholar
|
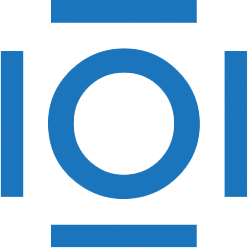
CITATIONS
Cited by 5 scholarly publications.
Nd:YAG lasers
Pulsed laser operation
Dye lasers
Tissues
Continuous wave operation
Laser sources
Mode locking